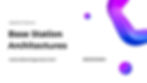
Standalone Base Station
Introduction to Standalone Base Stations
A 5G Base Station is known as a gNode B (next 'generation' Node B). This is in contrast to a 4G Base Station which is known as an eNode B ('evolved' Node B), and a 3G Base Station which is known as a Node B.
Architectures of Standalone Base Stations
Figure 21 illustrates two Standalone (SA) Base Station architectures, known as 'option 2' and 'option 5'. These names originate from the 3GPP study of 5G radio access technologies documented within 3GPP Technical Report 38.801. Both architectures have Base Stations that connect to the 5G Core Network.
Option 2 Architecture
The 'option 2' architecture is based on a gNode B connected to the 5G Core Network. The gNode B uses the New Radio (NR) air interface and signaling protocols towards the end-user device. It connects to an Access and Mobility Management Function (AMF) for control plane signaling and to a User Plane Function (UPF) for the transfer of application data. The gNode B and AMF communicate using the Next Generation - Control Plane (NG-C) interface, which employs the Next Generation Application Protocol (NGAP) to transfer signaling messages.
These messages are specified within 3GPP TS 38.413 and are transferred using SCTP over IP.
The gNode B can be connected to one or more AMFs and selects an initial AMF for each User Equipment (UE). The UE and AMF signal to one another using Non-Access Stratum (NAS) signaling messages, which are transferred between the gNode B and AMF via NGAP. Some specific types of application data, such as SMS content, can be transferred using NAS signaling messages.
Option 5 Architecture
The 'option 5' architecture is based on a next-generation eNode B (ng-eNode B) connected to the 5G Core Network. This involves an upgraded 4G Base Station connected to the 5G Core Network. The ng-eNode B uses the E-UTRA (4G) air interface and signaling protocols towards the end-user device. It connects to the 5G Core Network using the NG-C and NG-U interfaces and is capable of supporting 5G core network signaling procedures and transferring application data to and from a User Plane Function (UPF).
Non-Standalone Base Station
Introduction to Non-Standalone Base Stations
Non-Standalone (NSA) Base Stations use Multi-RAT Dual Connectivity (MR-DC) to provide user plane throughput across both the 4G and 5G air interfaces. This requires an eNode B and gNode B to operate together, connected using non-ideal backhaul, i.e., a realistic transport connection. Both the eNode B and gNode B have their own independent packet schedulers for allocating resources to the UE.
Legacy 4G UEs can continue to use the eNode B in the normal way, while newer UEs with 4G/5G capability can take advantage of the Multi-RAT Dual Connectivity. NSA Base Stations can provide an evolution path from 4G to 5G.
Configurations of Non-Standalone Base Stations
Figure 22 illustrates two configurations for Non-Standalone Base Stations using the 4G Core Network. These configurations, known as 'option 3' and 'option 3a', can be deployed before introducing the 5G Core Network.
Option 3 Configuration
In 'option 3', the 4G eNode B provides control plane connectivity towards the Core Network and acts as the Master Node (MN), while the 5G gNode B acts as the Secondary Node (SN).
The gNode B supports the X2 interface rather than the Xn interface. A drawback of this configuration is that all user plane data is tunneled through the legacy eNode B, which may not support the higher throughputs offered by 5G.
Option 3a Configuration
Option 3a addresses the drawback of option 3 by providing user plane connectivity between the S-GW and gNode B. The eNode B remains the Master Node and controls the selection of the downlink data path from the S-GW. However, this configuration does not support the transfer of application data across the X2 interface, which may be necessary if a UE moves out of the gNode B coverage area.
Advanced Non-Standalone Base Station Architecture
A third NSA Base Station architecture, known as 'Option 3x', is illustrated in Figure 23. In this configuration, the user plane paths are fully meshed between the eNode B, gNode B, and S-GW. The eNode B remains the Master Node and controls the downlink data path selection from the S-GW. If coverage from the gNode B becomes weak, data can be dynamically forwarded across the X2 interface to the eNode B.
EN-DC and UE Requirements
The deployment options illustrated in Figures 22 and 23 are known as E-UTRA New Radio Dual Connectivity (EN-DC), where E-UTRA refers to the 4G air interface and New Radio refers to the 5G air interface. UE supporting EN-DC must be capable of receiving downlink data simultaneously across the 4G and 5G air interfaces.
The 3GPP Release 15 specifications define EN-DC band combinations, including up to 5 different operating bands. Band combinations can include a mix of FDD and TDD operating bands. Generally, UE supporting EN-DC must be capable of transmitting uplink data simultaneously across the 4G and 5G air interfaces. However, some exceptions are allowed due to potential issues with inter-modulation products.
Signaling Procedures
The eNode B is the Master Node, so most RRC signaling procedures terminate at the eNode B. Signaling Radio Bearers (SRBs) such as SRB0, SRB1, and SRB2 terminate at the eNode B, with the 4G RRC signaling protocol specified in 3GPP TS 36.331 being applicable. SRB3 can be set up at the request of the Secondary Node (gNode B) and terminates at the gNode B, using the 5G RRC signaling protocol specified in 3GPP TS 38.331.
In conclusion, both Standalone and Non-Standalone architectures provide flexible and scalable solutions for the deployment of 5G networks, catering to different stages of the transition from 4G to 5G and various network requirements.
User Plane Bearer Types in EN-DC Base Station
These are Master Cell Group (MCG) Bearer, Secondary Cell Group (SCG) Bearer and Split Bearer.
Master Cell Group (MCG) Bearer
An MCG Bearer uses the RLC, MAC and Physical layers within the Master Node, i.e., it uses the 4G implementation of these layers and the 4G air-interface.
The PDCP layer is located within the Master Node if the SI connection is between the S-GW and the cNode B.
This represents a special case because the PDCP layer can be configured to operate in either a 4G or 5G mode. Some services may benefit from using the 4G mode while other services may benefit from using the 5G mode.
The 4G version of the PDCP layer (3GPP TS 36.323) supports a minimum Sequence Number (SN) length of 7 bits. The 5G version of the PDCP layer (3GPP TS 38.323) supports a minimum Sequence Number length of 12 bits. The sequence number is included within the PDCP header information.
The speech service can benefit from the shorter Sequence Number length because it helps to minimise the overhead generated by the PDCP layer. Thus, user plane bearers transferring speech content may be allocated an MCG bearer using the 4G PDCP layer. These bearers would then be kept on the 4G network and use only the 4G air-interface.
The data service typically uses larger packets so the percentage overhead generated by the PDCP layer is smaller. This means that larger Sequence Number sizes can be used without having a significant impact. In addition, it is necessary to allocate an increased number of bits to the Sequence Number when using high throughput applications. This provides a larger set of Sequence Numbers and helps to avoid issues associated with Sequence Number ambiguity.
User plane bearers transferring data content may be allocated an MCG bearer using the 5G PDCP layer. This could be applicable to an end-user located outside of 5G coverage, i.e., the MCG bearer uses only the 4G air-interface. If the end-user subsequently moves into 5G coverage then the MCG bearer using the 5G PDCP layer can then be switched to a Split bearer without having to reconfigure the PDCP layer.
Secondary Cell Group (SCG) Bearer
A SCG Bearer uses the RLC, MAC and Physical layers within the Secondary Node, i.e., it uses the 5G implementation of these layers and the 5G air-interface.
SCG Bearers always use the 5G version of the PDCP layer irrespective of Master/Secondary Node.
Split Bearer
A Split Bearer uses the RLC, MAC and Physical layers within both the Master and Secondary Nodes, i.e., both 4G and 5G air-interfaces can be used.
Split Bearers always use the 5G version of the PDCP layer irrespective of the node providing the SI connection towards the S-GW. This is known as a 'unified' solution because the location of the SI connection is transparent to the UE and both Split Bearers appear to be the same from the perspective of the UE.
Non-Standalone Base Station Configurations Using the 5G Core Network
These configurations are known as 'option 4' and 'option 4a' within the context of the 3GPP RAN architecture evaluation process (3GPP TR 38.801).
In both cases, the SG gNode B provides control plane connectivity towards the Core Network and acts as the Master Node (MN).
The Next Generation eNode B (ng-eNode B) has control plane connectivity across the Xn interface and acts as the Secondary Node (SN). These configurations require the ng-eNode B to support the Xn interface rather than the X2 interface.
In the case of 'option 4a', the ng-eNode B also has to support the NG-U interface towards the User Plane Function (UPF). Neither option requires the ng-eNode B to connect to the Access and Mobility Management Function (AMF).
The deployment options illustrated in Figure 26 are known as New Radio - E-UTRA Dual Connectivity (NE-DC), where New Radio refers to the 5G air-interface and E-UTRA refers to the 4G air-interface.
User Plane Bearer Types in NE-DC Base Station
These are similar to those supported by an EN-DC Base Station but with some important differences.
All user plane bearers connected to the SG Core Network use the Service Data Adaptation Protocol (SDAP) layer. This layer is located above the PDCP layer and is used to map QoS Flows onto Data Radio Bearers (DRB). The SDAP layer is not present when user plane bearers are connected to the 4G Core Network.
All user plane bearer types use the SG version of the PDCP layer. This is in contrast to the EN-DC configurations which are able to use either the 4G or SG version of the PDCP layer for MCG Bearers hosted by the Master Node. Using the 5G PDCP layer for all user plane bearers simplifies the architecture and the UE implementation.
Non-Standalone Base Station Configurations Using the SG Core Network
These configurations are known as 'option 7' and 'option 7a'.
In both cases, the Next Generation eNode B (ng-eNode B) provides control plane connectivity towards the Core Network and acts as the Master Node (MN).
The gNode B has control plane connectivity across the Xn interface and acts as the Secondary Node (SN). These configurations require the ng-eNode B to support the NG-C, NG-U and Xn interfaces.
The deployment options illustrated in Figure 28 are another variant of E-UTRA - New Radio Dual Connectivity (EN-DC), where E-UTRA refers to the 4G air-interface and New Radio refers to the 5G air-interface. They are similar to options 3 and 3a but the 4G Core Network is changed to the 5G Core Network and the X2 interfaces become Xn interfaces.
CU-DU Split Base Station
The Centralised Unit (CU) Distributed Unit (DU) Split Base Station architecture allows the gNode B to be deployed using two physically separated units. These two units are connected using an open interface standardised by 3GPP, i.e., it should be possible to use a CU provided by one network vendor and a set of DU provided by another network vendor.
Centralised Unit (CU)
The CU provides support for the higher layers of the protocol stack. There is a single CU for each gNode B. The Centralised Units belonging to multiple gNode B can be implemented using a shared hardware platform. Cloud computing and Network Function Virtualisation (NFV) can provide benefits when deploying the population of CU.
Distributed Unit (DU)
The DU provides support for the lower layers of the protocol stack. There can be multiple DU connected to each CU. For example, there could be more than 100 DU connected to a specific CU. The DU includes both baseband processing and RF functions.
The general structure of a CU/DU Split base station architecture is illustrated in Figure 29. The CU supports the SDAP, RRC and PDCP protocol stack layers, whereas the DU supports the RLC, MAC and Physical layers. The SDAP layer will not be present if the CU is connected to a 4G Core Network.
CU-DU Split Base Station
The interface connecting the CU and DU is referred to as the F1 interface. The protocol stacks for the F1-U (user plane) and F1-C (control plane) interfaces are illustrated in Figure 30.
The GTP-U protocol is used for transferring user plane data across the F1-U interface.
The end-to-end delay between the CU and DU needs to be low to minimise the impact of transferring data between the PDCP layer and the RLC layer. The delay is typically less than 10 milliseconds.
CU-DU Split Base Station Architecture
The CU-DU Split base station architecture provides benefits in terms of deployment flexibility and performance.
CP-UP Separation
The gNode B can be further split into separate control plane and user plane functions. The E1 interface is used for transferring control plane signalling between these functions.
The SDAP and PDCP layers are part of the user plane function and the RRC layer is part of the control plane function. The RLC, MAC and Physical layers are included in the user plane function if a CU/DU Split architecture is not being used.
The CP-UP separation provides an efficient mechanism for scaling the network. For example, the control plane processing capacity can be scaled independently of the user plane processing capacity. This is likely to provide benefits if the gNode B is serving a large number of small cells or beams. This is a typical deployment scenario for a 5G gNode B.
The control plane function can be deployed within a centralised location and the user plane functions can be distributed at the network edge to minimise the delay when transferring user plane data. This is known as a Centralised-RAN or C-RAN deployment.
CP-UP Separation Architecture Antenna Architectures
The 5G Base Station uses a set of antennas that connect with the distributed unit. These antennas can be implemented using a passive or active architecture.
Passive Antennas
These are connected to the Base Station cabinet using feeder cables. The Base Station cabinet includes the transceiver and RF processing functions. The RF processing functions include filtering and amplification.
The RF processing functions can include a Mast Head Amplifier (MHA) to improve the uplink Signal to Noise Ratio (SNR). The downlink SNR is improved by increasing the RF transmit power.
Active Antennas
Active antennas include transceivers integrated into the antenna. This allows more transceivers to be used to support an increased number of beams when compared with a passive antenna.
The additional beams can be used to increase the sector capacity, improve the average received SNR and maximize the coverage.
Active antennas can be used with both Frequency Range 1 (450 MHz to 6 GHz) and Frequency Range 2 (24.25 GHz to 52.60 GHz). They are essential for the efficient implementation of Frequency Range 2.
Base Station Measurement Requirements
The 5G Base Station is subject to a set of conducted and radiated measurement requirements. These requirements are illustrated in Figure 32.
Conducted Requirements
These are measured at the Base Station cabinet or antenna subsystem.
The Base Station cabinet is a single unit that includes both the RF functions and the baseband processing functions. The antenna subsystem connects with the antenna and includes the RF functions but excludes the baseband processing functions.
The conducted requirements include in-band and out-of-band emissions.
Radiated Requirements
These are measured at the Radiated Interface Boundary (RIB).
The Radiated Interface Boundary is the point at which the transmitted and received signals are free-space signals rather than conducted signals. This is also referred to as the Far Field.
These requirements include spurious emissions and the radiated transmit power.